Audiology Masking – AC and BC | Interaural Attenuation | Dilemma: Assuming, you know, sounds that are presented which arrive at the right ear are played in the right ear, and that Sounds that are presented via the left ear are perceived by the left ear. But this is not the case at all. It is often, the sound being presented the sound being picked up by one ear is being processed by the opposite ear. To prevent confusion it is common to refer to the ear being tested as the test ear (TE) and to ring the other ear that is one the nontest ear (NTE), the ear not receiving a test. This Article outlines why this happens, how it is detected, and how the NTE is removed from the test.
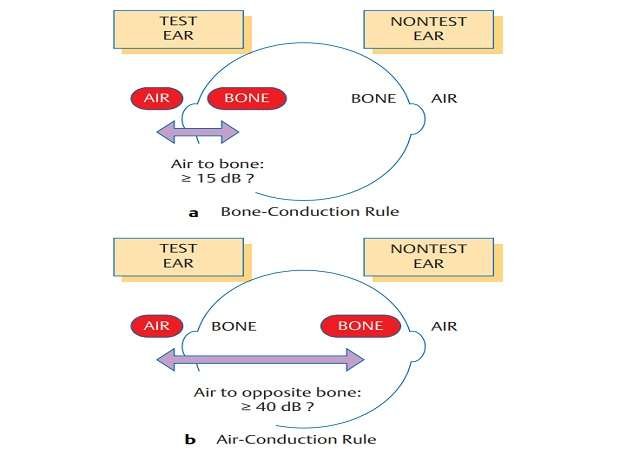
Table of Contents |
1. Audiology Masking 2. Purpose of Masking in Audiology 3. Cross Hearing 4. Interaural Attenuation 5. Selection of Masking Noises 6. Masking Rules in Audiology 7. Air Conduction Masking 8. Bone Conduction Masking 9. Speech Recognition Threshold (SRT) Masking 10. Audiology Clinical Masking Techniques 11. Masking Dilemma in Audiology 12. Case Studies for Audiology Masking by Air Conduction (AC) and Bone Conduction (BC) 13. Audiology Masking Cheat Sheet 14. Summary: Audiology Masking – AC and BC | Interaural Attenuation | Dilemma 15. FAQs related to Audiology Masking – AC and BC | Interaural Attenuation | Dilemma |
Audiology Masking
Masking in audiology is a tool that is used during hearing evaluations to guarantee the exact measurement of a person’s hearing thresholds. This means adding noise to the non-test ear to make it unable to hear a sound presented to the test ear. This is important because in some situations — specifically pure-tone audiometry, speech-recognition testing, and site-of-lesion testing — sound can “cross over” (or cross the head) from the test ear to reaching the nontest ear resulting in sound reaching a nontest ear, which introduces errors in results.
Audiometric testing involves the presentation of sound stimuli, often involving air conduction or bone conduction, and masking is critical to prevent the non-test ear from responding to the sound intended for the test ear. One technique employed by audiologists is applying masking noise to the non-test ear, isolating the test ear’s response for more accurate and reliable hearing assessments.
Purpose of Masking in Audiology
Masking is an important audiological process that allows for the determination of a more accurate hearing threshold by preventing the non-test ear from participating in the perception of the signal presented to the test ear. It guarantees accurate measurement of auditory function in patients with asymmetric hearing loss, bone-conduction testing, and speech recognition testing. Masking is done for several key reasons:
- Cross-Hearing Prevention: Sound presented to the test ear should not excite the non-test ear.
- Improves Accuracy: Provide a pure-tone response by isolating the test ear.
- Eliminates False Response: Prevents the cross-hearing adequately by preventing false responses.
- Necessary for Bone Conduction Testing: Because the same hearing pathways involve bone conduction to both ears, masking is crucial in determining true hearing differences in the ears.
- Used in Speech Recognition Testing: Only one ear response achieved, avoiding the non-test ear help in recognition of speech stimulus.
- Helps In Diagnosing Asymmetrical Hearing Loss: When one ear is much better than the other, it tries and find out the actual degree of hearing loss.
- Supports Site-of-Lesion Testing: Heeds test ear responses to maintain accuracy by identifying neural or cochlear pathologies.
Clinical masking must be used as needed/as possible in the following situations:
- Air conduction pure tone testing
- Bone conduction pure tone testing
- Speech Audiometry
Cross Hearing or Shadow Hearing
In this article to assume if sounds are presented at the right ear then they must be heard at the right ear, and the same for sounds to the left ear. But that is not always the case. Indeed, it is common for the sound presented to one ear to arrive at the opposite ear. This is known as Cross-Hearing or Shadow Hearing. To avoid confusion it is common to refer to the ear being tested as the Test Ear (TE) and the opposite ear, the ear not being tested as the Nontest Ear (NTE).
Signal Crossover and Shadow Curve
If a person does not hear any tone in the left ear during a test for air conduction, the intensity of the tone contributed to the left ear will be increased more and more. Ultimately, the tone sent to the hearing-impaired ear will reach a volume level so high that it will also be perceived in the nonaffected ear when finally we can expect the patient’s response to occur. The patient’s response to the signal presented to his deaf ear (the TE) is based on hearing that signal in the opposite ear (the NTE). Therefore, the threshold curve for the left ear shown in the Figure is a cross-hearing curve and is frequently referred to as a Shadow Curve.
Because the tone must be able to be heard in the NTE, a signal that is presented to one ear must be able to cross the head to reach the other ear. This effect is known as Signal Crossover.
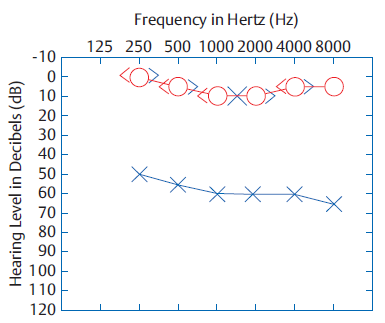
Interaural Attenuation
When the sound signal reaches the NTE, its intensity is lower than that given to the TE, because the signal requires energy to cross the head. All dB lost in the process of crossover from ear to ear are called Interaural Attenuation (IA) (Chaiklin 1967).
In the above figure, the patient’s right air conduction threshold at 1000 Hz is 10 dB HL. Even though his left ear is completely deaf, he also responded to a 1000 Hz tone presented from the left earphone at 60 dB HL. This means that the 60 dB HL tone presented in the left ear must have reached a level of 10 dB HL in the right ear. Consequently, IA at 1000 Hz in this case must be 50 dB (60 dB – 10 dB = 50 dB). Similarly, the amount of IA at 4000 Hz in this case is 55 dB (60 dB – 5 dB = 55 dB).
Interaural Attenuation for Air Conduction
Essentially high‐intensity auditory signals presented via earphones can “spill over” into the opposite ear (the non‐test ear). Early studies by Bekesy (1948) ascribed this effect to sound leaking around the earphone cushions and scattering around the head. Subsequent researchers reported that bone conduction (through pulsation of vibration at the skull) can transfer the sound to the contralateral cochlea as well. Consequently, the “crossover” of an air‐conducted test signal is possible within both around‐the‐head (air) and through‐the‐head (bone) pathways, with an estimated attenuation of ∼40 dB before it reaches the non‐test ear.
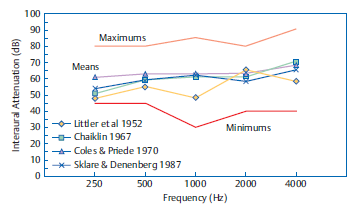
Interaural Attenuation for Air Conduction = 40 dB (hypothetically speaking for Supra-aural Headphones TDH-49 and related Receivers)
The only reported value of interaural Attenuation for air-conducted stimuli by any investigator is 40 dB. The interaural attenuation value for air-conducted stimuli that should be used clinically as the best option to reduce the likelihood of cross-hearing is 40 dB.
Interaural Attenuation for Bone Conduction
Vibrations traveling along the skull can create sound by directly stimulating the cochlea, bypassing the outer ear and middle ear. Bone conduction operates through the interconnected bones of the skull. At the cochlea, the phase-shifted bone-conducted signals can in fact cancel the air-conducted ones, they found.
There are three main types:
- Distortional: Vibrations compress the skullbones, squeezing the inner ear fluids to produce a traveling wave.
- Inertial: The ossicles (tiny ear bones) follow the movement of the skull, which displaces the stapes in the inner ear.
- Osseotympanic: Vibrations transfer energy to the external ear’s bone and cartilage, stimulating the tympanic membrane and enhancing low-frequency sounds.
Although the right ear is normal, the left one is deaf, so the right bone-conduction threshold and the left bone-conduction threshold are the same in the above figure. This implies the signal based on bone conduction transmitted to the left of the head reaches the right ear. It probably shouldn’t surprise you that a bone-conduction vibrator excites both cochleae roughly equally. From the perspective of cross-hearing, we can consider no interaural attenuation (IA = 0 dB) for bone conduction. The right and left bone-conduction signals, therefore, yield identical thresholds, since both are stimulating the same (right) ear.
Interaural Attenuation for Bone Conduction = 0 dB (hypothetically speaking)
IA for the more conventional mastoid placement of the bone-conduction oscillator varies, depending upon the frequency being tested, and also varies across patients (Studebaker 1964, 1967). The interaural attenuation for bone-conduction signals presented at the mastoid is ~ 0 dB at 250 Hz and increases to ~ 15 dB at 4000 Hz (Studebaker 1967). The author’s experience corroborates others’ clinical findings that the IA for bone conduction differed from patient to patient, ranging approximately from 0 to 15 dB at 2000 and 4000 Hz (Silman & Silverman 1991).
Interaural Attenuation for Insert Earphones
In contrast as shown on the figure, insert earphones (for example Etymotic ER-3A and EARtone 3A receivers) provide vastly increased amounts of IA (Killion, Wilber, & Gudmundsen 1985; Sklare & Denenberg 1987). The reason is that IA is inversely related to the contact area between the earphone and the head (Zwislocki 1953), and the contacts area between the head and earphone is much less when using insert receivers than when using supraural earphones. Some of the results obtained by Sklare and Denenberg (1987) in a comparison of IA from TDH-49 (supra-aural) and ER-3A (insert) earphones are displayed in the figure above, with testing done on the same subjects. For insert receivers, they reported mean IA values ranging from 81 to 94+ dB up to 1000 Hz and from 71 to 77 dB at higher frequencies.
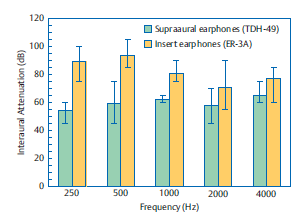
Interaural Attenuation for Insert Earphone = 70 dB (hypothetically speaking for Insert Earphones ER-3A and related Receivers)
We already noted that we care about the minimum IA values that the error lines of the graph represent which are where do each curve hits the bottom. Insert receivers, according to Sklare and Denenberg, yielded the lowest IA values at frequencies of up to 1000Hz (75 to 85 dB) and from 1000Hz and up (50 to 65 dB). This is much higher than the low IA values for TDH-49 earphones, which were 45 to 60 dB.
Selection of Masking Noises
What kind of noise should be used to mask the nontest ear? The answer to this question varies with the signal that is being masked. If the masked signal is wide-band, e.g. speech or clicks, the masker also must be wide-band, whereas for narrow-band signals, like pure tones, the masker must also be narrow-band.
Masking Noise for Pure Tone Audiometry (PTA)
Wide-band noises can also conceal pure tones, but this is not ideal. This however suggests that in the case of pure tones, Narrow-band Noise (NBN) would be the best masking noises.
- Narrow-band Noise (NBN): The actual masking noise bandwidths offered by audiometers are wider than critical bands. Advancement on this variation of masking noise is known as narrow-band noise (NBN).
The auditory frequency NBN may be close approximations of a one-third octave, one-half octave, or other-pass filter, and vary considerably with respect to the roll-off of intensity outside the pass band (i.e., the rejection rate or steepness of the skirts of the pass filter). So we can call it a 1000 Hz NBN if it is centered around 1000 Hz, a 2000 Hz NBN if it is centered around 2000 Hz, and so forth.
The table summarizes the bandwidths for narrow-band masking noises specified by the ANSI S3.6-2010 standard.
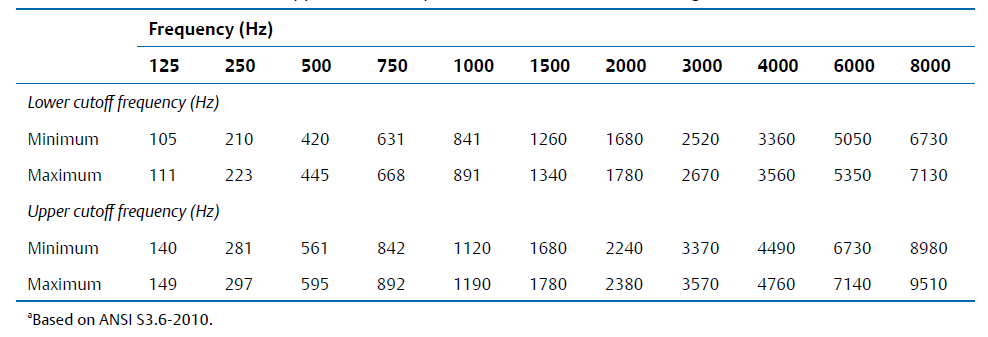
Masking Noise for Speech Recognition Threshold (SRT)
If the masked signal is wide spectrum (e.g., speech, clicks), the masker will also need to be the wide spectrum.
- Wideband/Broadband noise (white noise): Equal amount of all frequencies throughout the audible sound spectrum, often described as hissing, static-like sound
- Pink noise: Random noise with equal energy per octave, and thus containing morelow-frequency components than white noise.
- Speech-shaped noise: Speech-shaped noise has a spectrum that is similar to the long-term spectrum of speech.
- Multi-talker babble: Multitalker babble consists of recordings of many individuals speaking at once to create an unintelligible babble.
Masking Rules in Audiology
Masking rules must therefore be applied to ensure that masking noise will be correctly introduced into the non-test ear (i.e., in which hearing is not being tested) when testing the test ear (i.e., the ear being tested). The main goal of these rules is to analyze the possibility of cross-hearing so that it losing the correctness in the auditory assessment can be avoided. Therefore, implementing masking appropriately is critical for ensuring reliable and valid testing outcomes during testing.
When to Mask for Air Conduction (AC)
Air Conduction Masking Rules: The rule for air-conduction masking is demonstrated in the figure, and it can be stated in various ways. Based on the IA minimum value of 40 dB, AC will need to be masked whenever there is a difference of 40 dB or greater between the test ear’s air-conduction threshold ACTE and the nontest ear’s bone-conduction threshold BCNTE. Some audiologists refer to the spread between ACTE and BCNTE as an air-opposite-bone-gap (AOBG) or an air-contralateral-bone-gap (ACBG). With this terminology, masking is needed if whatever of relationship holds:
AOBG ≥ 40 dB
For the numerically inclined, an air-conduction threshold must be retested with masking whenever:
(ACTE − BCNTE ) ≥ 40 dB
When to Mask for Bone Conduction (BC)
Bone Conduction Masking Rules: Bone-Conduction Masking Rules: An individual’s bone–conduction threshold must be re-evaluated with respect to masking in the NTE whenever an airbone-gap (ABG) exists in the test ear that is greater than 10 dB, that is, 15 dB or larger, which may be expressed as:
ABG > 10 dB
As testing is performed in 5 dB steps, this rule can also be expressed in the following manner: Bone-conduction threshold should be re-tested with masking in the NTE whenever the air-bone-gap (ABG) for the test ear is 15 dB or greater or
ABG ≥ 15 dB
When to Mask for Speech Recognition Threshold (SRT)
Speech Recognition Threshold (SRT) Masking Rules: If the SRT of the test ear is greater than the bone-conduction threshold of the non-test ear by 40 dB or more, masking is required for SRT testing. Data were compared to bone conduction or air conduction thresholds at different frequencies (including 500, 1,000 and 2,000 Hz or others as applicable).
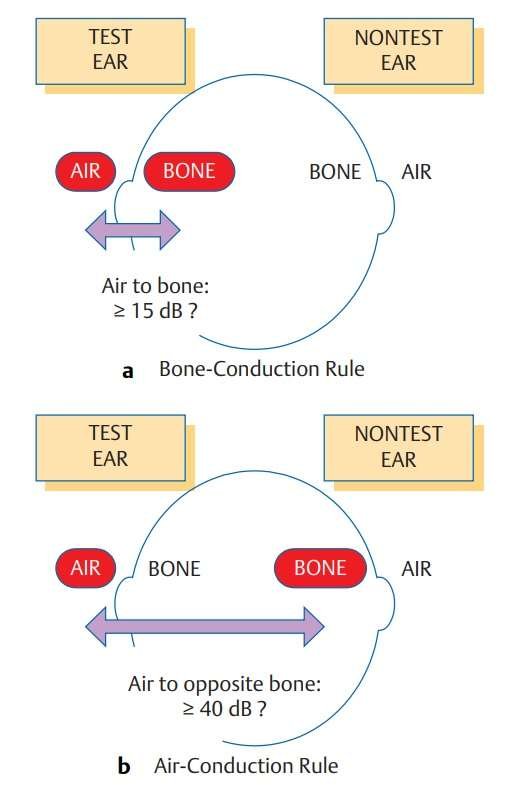
Air Conduction Masking
The masked air-conduction threshold is needed when the air-conduction threshold of the test ear is at least 40 dB below the true bone conduction threshold (or lower air-conduction threshold) of the nontest ear. Individual threshold values can be obtained by introducing masking noise into the nontest hearing ear. Because this process relies on awareness of the actual bone conduction thresholds, the bone-conduction masking must be performed before the air-conduction masking. The Air conduction threshold of the Left ear and the bone conduction threshold of the right ear difference is > 40 dB, so in this scenario, we require Air Conduction Masking for the left ear (Refer to Figure 1).
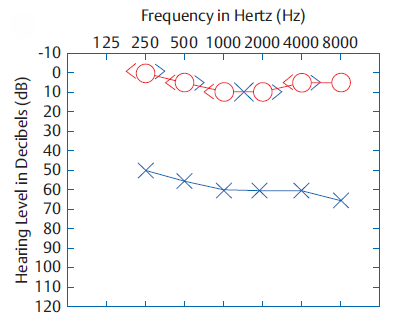
Air Conduction Masking Formulas
Minimum Effective Masking Correction (MEMC): The difference in decibels between the level of a particular tone and the noise level that just masks that tone. This difference is commonly referred to as the effective masking level (EML), but is potentially more clearly communicated by referring to it as the minimum effective masking correction (MEMC) because it is the correction that must be added to the level of the tone to end up with a minimum noise level that will effectively mask that tone.
To illustrate, an example might be that 55 dB HL of NBN masks a 50 dB HL tone just barely. This implies that the noise must be 5 dB greater than the tone to mask it; ie, the MEMC here is 55 dB – 50 dB = 5 dB.
Safety Factor (SF): This assumes we already know that our average minimum effective masking correction for our audiometer is 5 dB greater than the hearing level of the test tone for each frequency. In other words, the loudness of the masking noise must be 5 dB louder than the tone level.
Thus, a noise of 15 dB HL would be required to mask a tone at 10 dB HL, or 60 dB HL to mask a tone at 55 dB HL. And since the MEMC is an average, we should add a 10 dB safety factor as well. If we add a 10 dB safety factor, our starting masking level will correspond to an attenuator (dB HL) dial reading that is 15 dB higher than the unmasked threshold in the NTE.
Initial Masking Level Formula (IML) for Air Conduction (AC): For those mathematically inclined, the initial masking level (in dB HL) that is put into the NTE can be simplified to the equation:
IML = ACNTE + MEMC + SF
For Example:
20 dB HL (AC threshold of the tone we want to mask)
+ 5 dB (MEM correction)
= 25 dB HL (Average MEM level for a 35 dB HL tone)
+ 10 dB (Safety factor)
= 35 dB HL (Initial Masking Level)
Maximum Masking Level (MM) Formula for Air Conduction (AC): Maximum masking during Air Conduction is determined using the following formula:
MM = BCTE + IA (40 for supra-aural headphones or 70 for insert earphones)
For Example:
20 dB HL (AC threshold of the tone we want to mask)
15 dB HL (BC threshold of the Test EAR)
+ 40 dB (IA for Supra-aural Headphones)
= 55 dB HL (Maximum Masking)
Bone Conduction Masking
When an air–bone gap greater than 10 dB exists, the threshold of bone conduction in the test ear must be determined, and masked. To do this, it involves the administration of a narrow-band noise on the non-test ear whilst measuring the threshold in the test ear. As seen in Figure 1 where the difference between air conduction threshold and bone conduction threshold of the left ear is greater than 10 dB, we need to perform bone conduction masking for left ear in this situation.
Why Aren’t Bone Conduction Thresholds Obtained at 8 kHz During Pure Tone Audiometry?
Transducer Limitation: Bone conduction transducers (such as Radioear B-71s or equivalents) are designed and calibrated to operate in the frequency range of approximately 250Hz to 4–5kHz. They are tuned to deliver reliable, quantifiable vibrations in this frequency range.
Skull and Tissue Damping: The skull’s physical structure is much less efficient at transmitting higher frequencies than the lower ones. So although a transducer might be able to produce an 8kHz signal, much of that energy is lost or changed when it reaches the cochlea.
Occlusion Effect
The external ear canal naturally acts as a resonant body, amplifying frequencies at around 2–4 kHz for its own shape (~2.5 cm long). When occluding, resonance properties change, resulting in enhancing of lower frequencies (125Hz to 1000 Hz), by 20–30 dB in many cases.
Meanwhile, the IML values for bone-conduction testing are calculated in a similar manner as that for air-conduction but additionally consider the factor of the occlusion effect (OE). This is to avoid the introduction of an occlusion effect by the earphone covering the NTE, which is the phenomenon where the level of a bone-conduction signal introduced into the ear canal is increased due to heading occluding the ear canal.
How to determine the Occlusion effect
Therefore, we need the OE size to calculate the IML. One method is to use fixed OE values (e.g., Studebaker 1979; Goldstein & Newman 1985; Yacullo 2009), as shown in the Table. This approach, which is advocated here, is that clinicians must ascertain whether and how much the OE, exists in that individual patient.
This is because we cannot assume any predetermined value due to the occlusion effect:
- differs with frequency,
- that is extremely susceptible to interindividual variability, and
- is missing in the presence of a conductive pathology
Thus, the presence and size of OE need to be confirmed with an easy but fundamental test before the application of IMLs for BC. The procedure recommended uses the audiometric Bing test (Martin, Butler, & Burns 1974) and is as follows:
- With unmasked bone-conduction thresholds already acquired, the audiologist places the earphone over the NTE and retests the bone conduction with no noise.
- An OE is said to exist if the occluded threshold is superior (lower) than the unoccluded threshold.
- The size of the OE is simply the difference between them.
For the example above, the unoccluded bone conduction threshold will be 35 dB and the occluded bone conduction threshold will be 20 dB HL thus showing that the OE = 35 – 20 = 15 dB. This OE value is then used to build the IML. When the OE is zero, the bone conduction IML formula is identical to the air-conduction formula. This is performed from frequencies starting with 1 Hz up to 1000 Hz, which is the frequency region where we expect OEs to happen.
Bone Conduction Masking Formulas
IML for bone conduction testing is very much like the air conduction IML with the critical exception being a factor for the occlusion effect (OE). The earphone covering the NTE introduces an occlusion effect, an apparent increase in the level of the bone conduction signal as a result of occluding the ear canal.
Initial Masking Level Formula for Bone Conduction (BC): The original OE-adjusted formula for the initial masking level during bone-conduction testing may be expressed as
IML = ACNTE + MEMC + SF + OE
For Example:
35 dB HL (AC threshold of the tone we want to mask)
+ 5 dB (MEM correction)
= 40 dB HL (Average MEM level for a 35 dB HL tone)
+ 10 dB (Safety factor)
= 50 dB HL (IML, unoccluded)
+ 15 dB (Correction for the OE)
= 65 dB HL (Initial masking level accounting for the OE)
Maximum Masking (MM) Level Formula Bone Conduction (BC): We find maximum masking in the Bone conduction testing by the following formula:
MM = BCTE + IA (40 for supra-aural headphones or 70 for insert earphones)
For Example:
35 dB HL (AC threshold of the tone we want to mask)
30 dB HL (BC threshold of the Test EAR)
+ 40 dB (IA for Supra-aural Headphones)
= 70 dB HL (Maximum Masking)
Speech Recognition Threshold (SRT) Masking
When the SRT of the test ear is greater than the bone conduction threshold of the nontest ear by 40 dB or more at any frequency, the masked speech-recognition threshold (SRT) is required.
Initial Masking Level Formula for Speech Recognition Threshold (SRT): This initial formula applied for speech recognition testing and incorporating the OE can be expressed as
IML = SRTNTE + MEMC + SF
For Example:
20 dB HL (SRT threshold of the tone we want to mask)
+ 5 dB (MEM correction)
= 25 dB HL (Average MEM level for a 35 dB HL tone)
+ 10 dB (Safety factor)
= 35 dB HL (Initial Masking Level)
Maximum Masking (MM) Level Formula for Speech Recognition Threshold (SRT): The formula for determining maximum masking during Speech Recognition testing is:
MM = BCTE + IA (40 for supra-aural headphones or 70 for insert earphones)
For Example:
20 dB HL (SRT threshold of the tone we want to mask)
15 dB HL (BC threshold of the Test EAR)
+ 40 dB (IA for Supra-aural Headphones)
= 55 dB HL (Maximum Masking)
Audiology Clinical Masking Techniques
The audiological masking techniques are precious clinical routines that help to obtain real hearing thresholds by isolating the test ear from a potential interference of the non-test ear. Around Audio assessments, and during subsequent evaluations, Implementing these techniques including Maximum Masking (MM), Central Masking, and the Plateau Method assists clinicians with dealing with and lessening cross-hearing. By carefully applying a masking noise to one ear, audiologists are more able to find the true hearing levels of the target ear, resulting in greater diagnostic accuracy and better treatment planning.
Instruction for Hearing Tests with Masking
In clinical masking, the first step is to let the patient know what to expect and to let her know what she needs to do. Even the idea of being tested with “noise in your ears” can confuse some patients, particularly if they are being tested for the first time. When patients are told that putting masking noise in the opposite ear is like an optometrist covering one eye when the other is checked, even the most argumentative has accepted this without argument, the author has found.
Maximum Masking
MM (Maximum Masking) is defined as the highest level of masking noise that can be introduced into the non-test ear without overmasking the test ear. The maximum masking is the maximum amount of maskin noise in dB HL that is not quite sufficient to produce overmasking of the test ear. The formula for calculating maximum masking in the contexts of bone conduction, air conduction, and speech-recognition testing is:
MM = BCTE + IA – 5 dB
where BCTE is the bone-conduction threshold of the test ear and IA is the interaural attenuation for air conduction (40 dB).
Central Masking
Central masking when a noise, which was added to one ear, affects the auditory threshold of a tone in the other ear. Even though the noise and tone are processed by separate cochleae, their neural signals converge in the CANS, which leads to interference. Central masking is thought to function differently than direct, ipsilateral masking—where both stimuli are presented in the same ear—with interaural interaction resulting in a shift in threshold even when no sound physically crosses over. This complicates masking in the clinic, because when this happens, the measured threshold in the test ear may be artificially elevated. Clinicians usually consider the effect to be on the order of 5 dB, but shifts of 15 dB or more have been recorded at high levels of noise. If we care about getting accurate auditory tests, we need to properly compensate for this central interaction so that only the ear we want to test is considered.
Key Points:
- Occurs with noise and tone in opposite ears.
- Involves neural convergence in the CANS.
- Typically produces a threshold shift of around 5 dB.
- Can complicate clinical masking, necessitating adjustments.
For example, if a tone is presented at 5 dB HL in the right ear and noise is also presented in the left ear simultaneously, central masking may cause the right ear’s threshold to shift from 5 dB HL to 10 dB HL.
The Plateau Method
One common approach to obtaining the true masked threshold of the test ear (TE)when using masking noise in the non-test ear (NTE)is the plateau method (also called the threshold shift method). The initial value of the TE (without masking) is obtained, and when masking noise is applied, the apparent threshold is displaced upwards. When the level of masking noise is raised, the resulting data normally fall into three regions: undermasking, where the noise is not enough to completely mask the tone; the plateau region, where the masked threshold is relatively fixed, indicating effective masking; and overmasking, where too much noise begins to mask out the tone altogether. Described by Hood in 1960, this method assists clinicians in accurately determining the TE’s threshold such that crossover hearing is effectively controlled during the course of testing.
Undermasking
If a tone is indeed being transmitted to the non-test ear via cross-hearing, then increasing the level of the masking noise will lead to an increase in the masked threshold. This happens because the noise and the tone share a (nontest) ear. For instance, the data plotted in the figure indicate that increasing the masking noise from 20 to 35 dB HL resulted in an increase of the apparent tonal threshold from 60 to 75 dB HL.
This linear increase of apparent threshold with masking noise level suggests that the tone is still being picked up by the NTE. We refer to this as undermasking, because the noise level is not high enough to mask the NTE from the test. So, undermasking is well defined when sufficient masking noise is not presented, where the tone is being perceived by the nontest ear like in Figure 2. a.
The Plateau Width
The plateau is said to occur when the NTE is sufficiently masked by the noise such that the tone is perceptible in the test ear. In this case, the tone is detected only in the test ear, while the noise is detected only in the non-test ear. If the tone is truly being heard in the test ear, then increasing the level of masking noise will not affect the threshold. This is shown in the central portion of Figure 1 and conceptually in Figure 2. b, where the threshold for the tone remains constant at 75 dB HL even though the masking noise is increased from 35 to 65 dB. This plateau shows the true masked threshold for the tone in the test ear. The horizontal extent of the plateau is sometimes referred to as the range of effective masking.
Overmasking
At some point, the noise intensity will become strong enough to cross over to the test ear. This will occur when the level of the masking is raised, which will then result in the threshold for the tone being raised. This region on the plateau plot is commonly referred to as overmasking, which is the diagonal line on the right side of figure 1 and also shown in figure 2. c. A more detailed discussion of overmasking will follow in a later section.
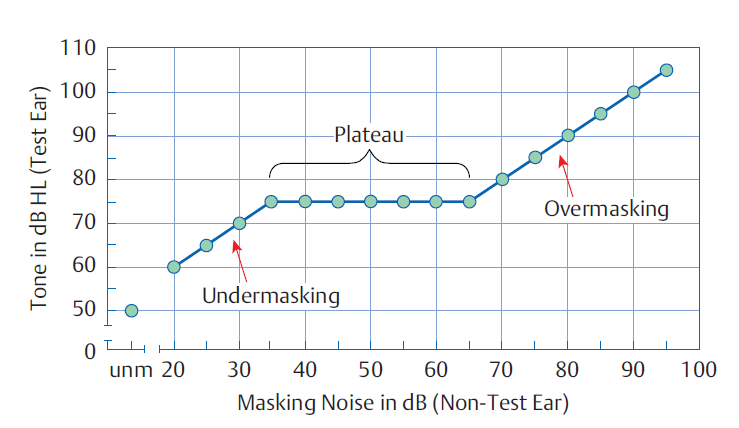
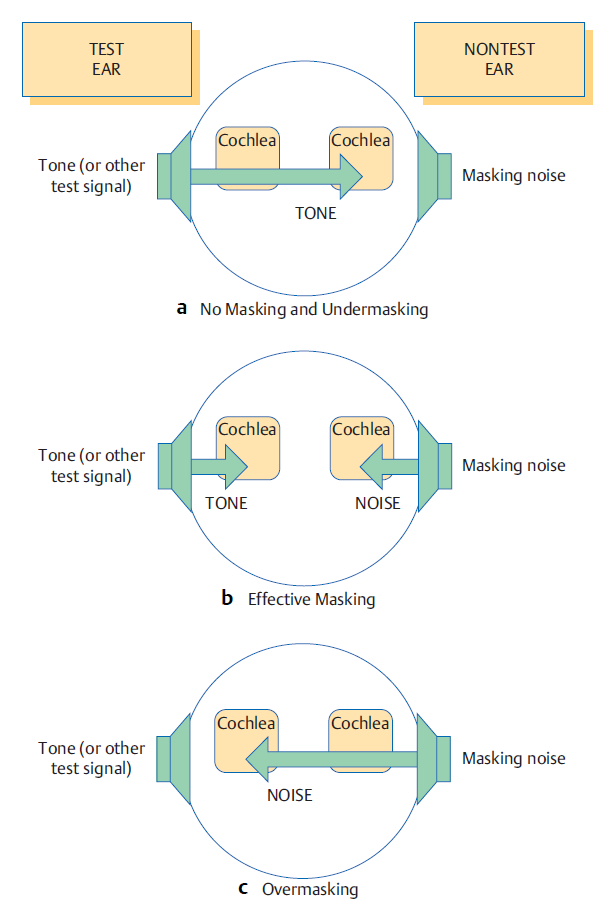
Masking Dilemma in Audiology
Indeed, initial masking (IM) can induce overmasking in certain contexts. Example: Unmasked air‐ and bone‐conduction thresholds for a single patient are presented in the Audiogram. Thus at 1,000 Hz, if the ME is 15 dB, and the occlusion effect is 10 dB, the initial masking level will be 50 dB (35 dB + 15 dB). Overmasking can occur when the level in the nontest ear is equal to or exceeds the bone-conduction threshold in the test ear + 40 dB HL. Here, with a test-ear bone-conduction threshold of 0 dB HL, you could overmask the test ear by adding 65 dB HL of masking. This leads to a worst case scenario should the true interaural attenuation (IA) be lower than the assumed value due to confusion over the occlusion effect vs a change in IA vs true overmasking.
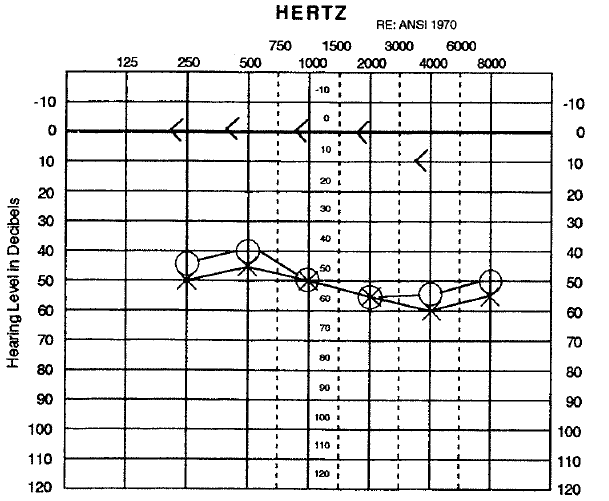
When overmasking does happen, there cannot be a plateau (i.e., a stable masked threshold) and it is ambiguous whether the measured threshold is authentically that of the test ear or simply the response of the nontest ear to the presented signal. This is particularly problematic for bilateral conductive losses or a significant air-bone gap, as the limiting factor of how much masking can be applied is the difference between the test-ear bone-conduction threshold and that for the nontest ear (plus any air-bone gap). This can lead to what is termed a “masking dilemma,” in which the level of masking needed either does not provide enough masking for the nontest ear or is considerable enough that it overmasks the test ear.
In addition, masking dilemmas occur less frequently when using Insert earphones because higher interaural attenuation of the masker will occur, so overmasking is less common, as IA for insert earphones is around 70 dB.
Case Studies for Audiology Masking by Air Conduction (AC) and Bone Conduction (BC)
Audiogram 1 shows the Air-conduction thresholds only for a hypothetical patient. These results were plotted during air-conduction testing.
Audiogram 2 shows the Air and Bone Conduction thresholds for the same patient as in Audiogram 1. Note that the mastoid bone-conduction thresholds for the left ear have been added to the audiogram. Audiogram 2 reveals the presence of significant air-bone gaps for the left ear of approximately 45 dB. Recall from the section on masking that the masked bone-conduction thresholds are obtained whenever there are air-bone gaps of 15 dB or more.
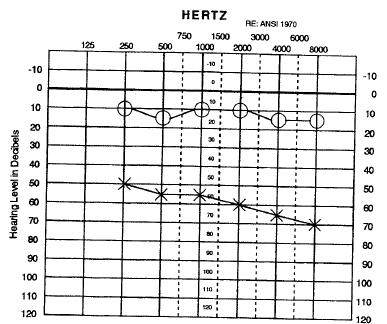
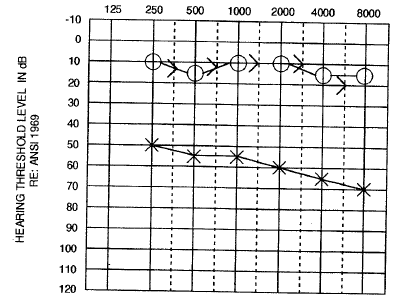
Sample Audiograms for Bone Conduction Masking
Audiograms 3, 4, 5, and 6 show four options for the masked left bone-conduction thresholds (there is more than four options) following a bone conduction masking. The left masked bone-conduction thresholds represent the bone-conduction threshold while the masking noise is routed through the earphone to the right ear and the bone vibrator to the left ear.
In Audiogram 3 – 6, the left masked bone conduction thresholds have been added to the unmasked air and bone conduction thresholds in Audiogram 2.
The masked left bone conduction thresholds in Audiogram 3 remain the identical as unmasked bone conduction thresholds exhibited in Audiogram 2.
The masked left bone-conduction thresholds in Audiogram 4, 5, and 6 are worse than the unmasked bone-conduction thresholds in Audiogram 2.
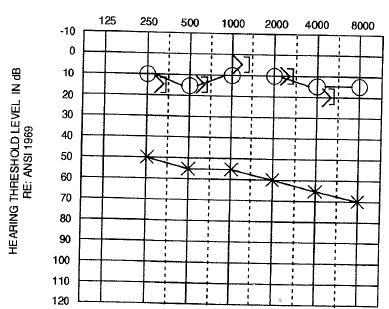
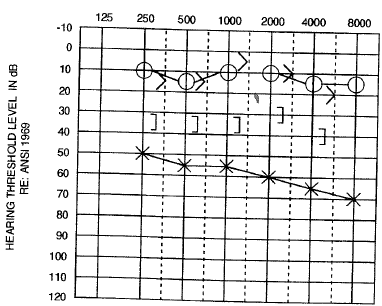
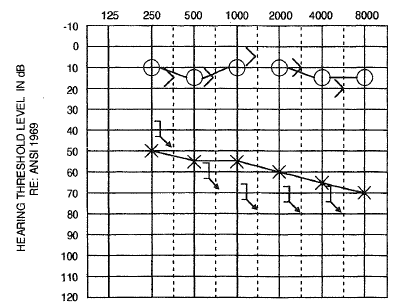
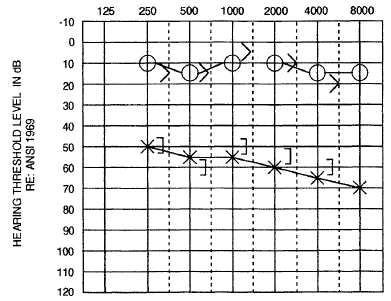
Sample Audiograms for Air Conduction Masking
The next step is that the clinician needs to infer if the masked Air conduction thresholds are required after the masked bone-conduction thresholds are obtained.
Audiograms 3 – 6 need to be analyzed for a difference of 40 dB or more between the bone-conduction threshold of one ear and the air-conduction threshold of the other ear.
If we look at Audiograms 3 – 6, we notice that masked air-conduction thresholds for the left ear must be attained in all cases, because the left unmasked air-conduction thresholds are 40 dB or more than the right bone conduction thresholds.
Audiograms 7, 8, 9, and 10 in particular present two, but not all, possibilities for the left masked air conduction thresholds, following masking for air conduction, depending upon the corresponding left masked bone conduction thresholds presented in Audiograms 3, 4, 5, 6, respectively. While recording the left air conduction thresholds, narrow-band noise is routed using the earphone to the right ear to generate the masked left AC thresholds.
In Audiogram 7, the masked left air conduction thresholds can be none lower than the unmasked left air conduction thresholds; the masked left air conduction thresholds can be worse than the unmasked left air conduction thresholds.
In Audiogram 8, the first options for the masked left air conduction thresholds are the same as the unmasked left air conduction thresholds; the second options for the left masked air conduction thresholds are worse than the left unmasked air conduction thresholds.
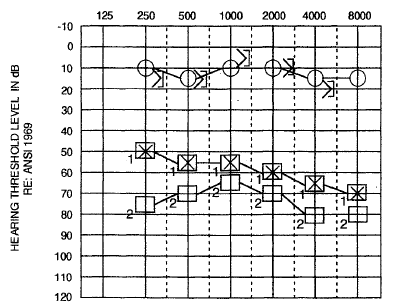
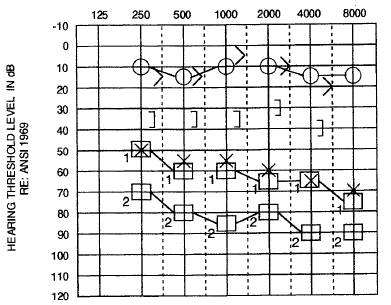
In Audiogram 9, both possible masked left air conduction thresholds are poorer than the unmasked left air conduction thresholds.
The first possibility for the masked left air conduction thresholds in Audiogram 10 are slightly worse than or equal to the unmasked left air conduction thresholds; the second possibility for the masked left air conduction thresholds are worse than the unmasked left air conduction thresholds.
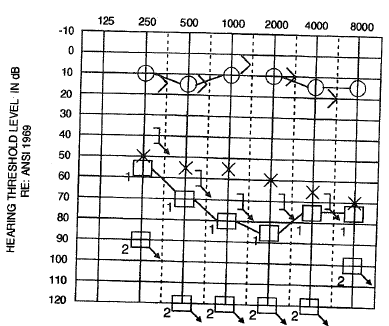
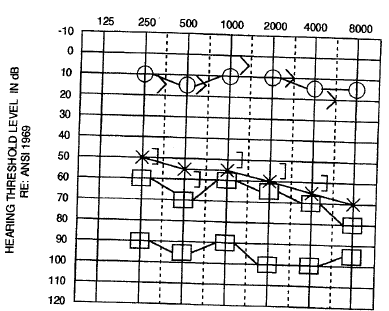
Notice that the masked air-bone gap (using each of the two possibilities for the masked left air-conduction thresholds) for the left ear (after air and bone conduction masking in Audiogram 7) is 35 – 60 dB and does not exceed the maximum air-bone gap limit of 60 dB discussed above in the masking portion of the text.
Audiology Masking Cheat Sheet
This audiology masking cheat sheet covers some terms and concepts used in transducer interactions (cross-hearing, interaural attenuation) and prescribes specific conditions for how to choose noise used in masking during pure-tone audiometry testing, speech recognition, and bone conduction testing. It details when and how to apply masking by following specific rules and formulas, such as the Initial Masking Level (IML) and Maximum Masking (MM), and describes clinical techniques like the plateau method for accurately determining true hearing thresholds without falling into undermasking or overmasking. The information presented in this cheat sheet educates the clinician to successfully navigate the complicated area of audiological masking.
Download Audiology Masking Cheat Sheet
Summary: Audiology Masking – AC and BC | Interaural Attenuation | Dilemma
It is performed when the test ear must be kept separate from the influence of the nontest ear during audiology testing. By introducing masking noise in the non-test ear, it avoids cross-hearing (detection of sound from one ear by the opposite ear). The process is crucial to pure-tone audiometry, bone conduction, and speech recognition assessments, it ensures hearing threshold measurements, such as in the diagnosis of asymmetrical hearing loss, and avoids false responses. Interaural attenuation is a measure of energy lost when sound travels across the head; these are in the order of 40 dB (air conduction) and 0 dB (bone conduction), but higher values can be measured with the use of insert earphones.
Masking rules utilize certain conditions (for example, an air-bone gap limit) and methods (for instance, plateau) in their audits. Specifically, this article describes a variety of masking equations, common clinical approaches, and potential dilemmas that may arise while applying masking in the testing of auditory function. It highlights the critical need for careful calibration and application to yield valid results.
FAQs related to Audiology Masking – AC and BC | Interaural Attenuation | Dilemma
In audiology, masking is a methodological practice applied to prevent the test signal from being heard by the ear that is not being tested, ensuring that responses only come from the ear of interest. In presenting controlled noise to the non-test ear, however, audiologists can prevent “cross-hearing” — when the sound could also be detected by the non-test ear, resulting in erroneous results. In the case of pure tone audiometry, if the right ear is tested, a noise masker is applied for the left ear. Isolating each ear during assessment improves diagnostic accuracy and helps identify type and degree of hearing loss.
For a given hearing test, masking in a hearing test refers to the noise that is introduced into the ‘non-test ear’ while presenting tones into the ’test ear’, so that the tones of the sound being heard in the opposite ear do not interfere with the tones. This becomes essential in the presence of asymmetric hearing loss, so it makes sure the sound will only processed by the target ear.
For example, if a patient has normal hearing in one ear and hearing loss in the other, the better ear might unintentionally register test tones. By masking the normal ear with sound, the audiologist can then measure the hearing threshold in the affected ear. Such a controlled approach aids with the reliability of the test and directs appropriate treatment.
In audiology, masking is indicated for significant asymmetry between the ears or when one ear has significantly better hearing sensitivity than the other. A test ear frequency prevents cross-hearing and results in the response being detected only from the test ear.
As an example, if a patient has normal hearing in one ear and hearing loss in the opposite ear if the test tones are not masked, the non-test ear can hear them and thereby, nonsensically, affect the results. Moreover, in unilateral hearing loss, masking is used for measuring pure tone thresholds to ensure that the obtained thresholds will represent the true hearing ability, which is vital for accurate diagnostics and effective treatment plans.
Audiologic masking is achieved through the application of noise to the non-test ear at the time of the hearing test. This involves choosing the right type and level of noise, usually broadband or narrowband, according to the patient’s hearing range.
Testing example: In pure tone audiometry, the audiologist presents a tone to the test ear while the non-test ear is set to a masking level. The masker is slowly increased until the ear not undergoing the test can no longer hear the tone. By using the probe to apply sound only to the ear being tested, this controlled application helps isolate the ear being tested so that no responses originate from the non-test ear, assisting the audiologist in obtaining accurate hearing threshold information from the patient.
This happens when a masking noise is presented at an intensity that encroaches upon the test ear, leading to an elevated artificial hearing threshold. This occurs to the extent that the noise being presented to the non-test ear “spills over” to the test ear and prevents detection of the test signals. During pure tone audiometry, if the masking noise level is too high, it may cause masking of the test ear as well which can give an erroneous picture of further hearing loss. Audiologists ensure that the masking noise only affects the non-test ear by carefully balancing and calibrating the level of the masker, as well as continuously monitoring the patient’s responses in order to provide adequate masking without over-masking.
References:
- Auditory Diagnosis Principle and Application – Shlomo Silman and Carol A. Silverman (Book)
- Introduction to Audiology – Frederick N.Martin (Book)
- Essentials of Audiology – Stanley A. Gelfand, PhD (Book)
You are reading about:
Audiology Masking – AC and BC | Interaural Attenuation | Dilemma
Please please it’s a request from a student please upload whole syllabus notes…
Because it’s very difficult to find BASLP notes anywhere…🥺
Please upload all topic notes of baslp, it’s difficult to find accurate notes on internet , please help us 🥺🥺🙏🙏🙏